Chromatic Chemistry: Transition Metals and Nature’s Hidden Color Palette
- Lori Preci
- Feb 24
- 4 min read
Nestled in the d-block of the periodic table, transition metals profoundly shape the vibrant world we see. While organic chemistry often captures the spotlight with its intricate molecules and reactions, the dazzling contributions of transition metals remain one of nature’s best-kept secrets. From the rich hues of gemstones to the molecular mechanisms of blood, these elements weave color into both the mundane and the extraordinary.
Nature’s Timeless Palette: A Brief History of Transition Metals
The story of transition metals intertwines with humanity’s enduring fascination with color. Their vivid hues have pervaded art and culture since antiquity.
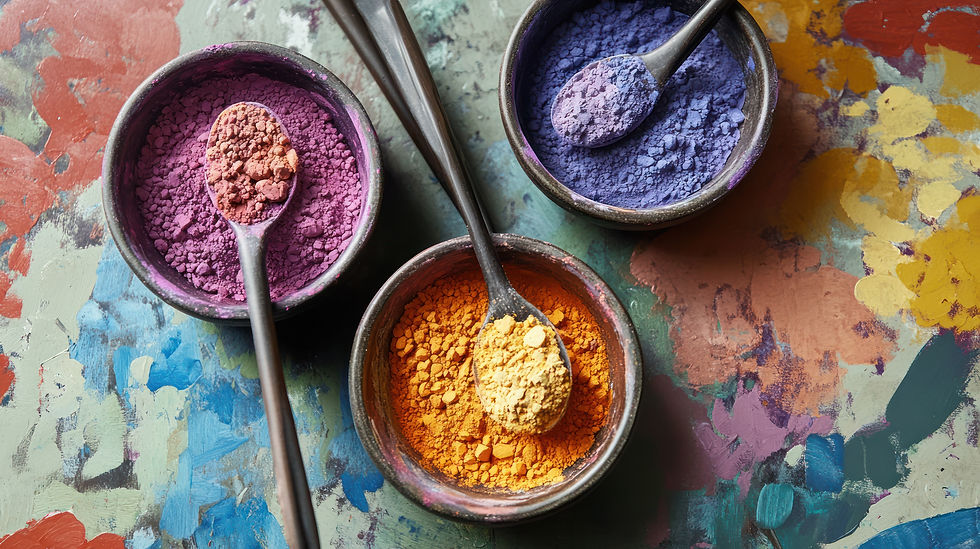
Long before synthetic dyes emerged, ochre stood out as an ancient earth-derived pigment. Composed of iron oxide, ochre’s color varies with its mineral form—from hematite-red (α-Fe₂O₃) to goethite-yellow (α-FeO(OH)). Archeological discoveries, such as the ochre-stained artifacts in Qafzeh Cave, Israel, suggest that early humans used these pigments in rituals as far back as 100,000 years. For ancient societies, ochre was more than a pigment, it symbolized life, death, and cultural expression.
Today, the legacy of transition metals lives on in art, whether in the copper greens of Renaissance paintings or the cobalt blues of stained glass. Their vibrant impact is as timeless as it is scientific.
Metallic Masterpieces: How Transition Metals Paint Biochemistry
Transition metals not only color our history but also animate the chemistry of life. Their secret lies in their partially filled d-orbitals, which allow these elements to interact with other molecules in unique ways. Transition metals can form coordination complexes, which occur when they bond with ligands—molecules or ions that donate a pair of electrons to the metal. This bonding changes the electronic structure of the metal ion, which in turn affects how it interacts with light. As light shines on a coordination complex, specific wavelengths are absorbed while others are reflected. The reflected light is what gives these complexes their brilliant colors.
Breathing Color: Hemoglobin and Hemocyanin
Transition metals are at the heart of respiratory pigments, making life possible. Hemoglobin, the oxygen-transporting molecule of our blood, binds oxygen via its iron-centered heme groups, imparting blood’s characteristic red hue. This hue shifts with oxygenation, appearing bright red when oxygen-rich and darker red when oxygen-poor.
Meanwhile other animals like horseshoe crabs owe their striking blue blood to hemocyanin, a copper-based oxygen carrier. Copper’s unique electronic interactions create this distinct hue, illustrating the versatility of transition metals in biochemistry.
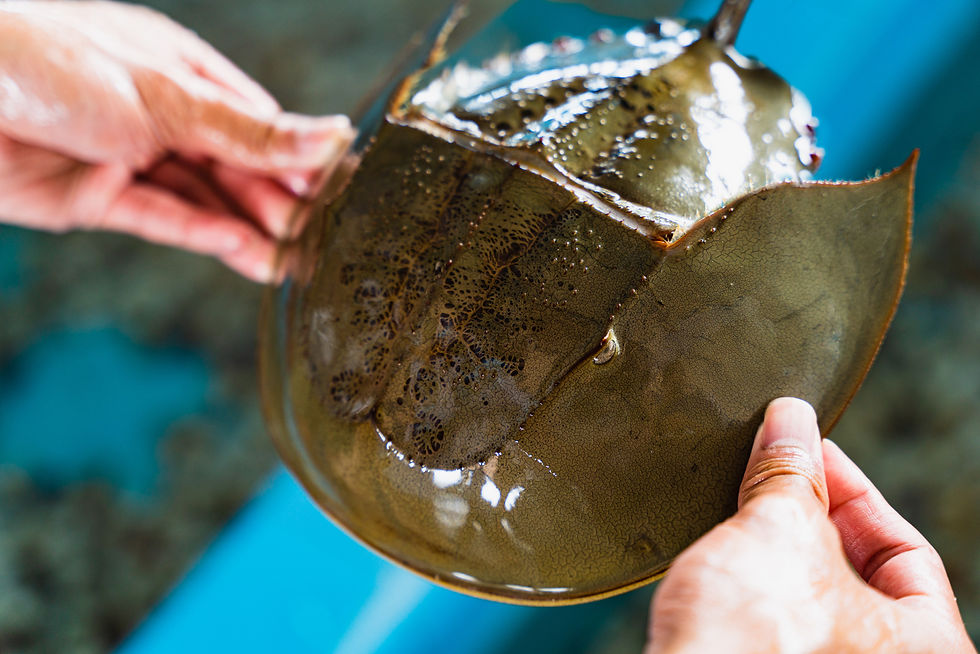
Decoding Crystal Field Splitting
The vibrant hues of transition metals are rooted in the behavior of their d-orbitals—regions around the metal ion where electrons reside. When ligands surround a transition metal ion to form a coordination complex, they create an electric field that influences the energy levels of the d-orbitals. Crystal Field Theory (CFT) provides a framework for understanding how the spatial arrangement of ligands around a metal ion perturbs the energy of its d-orbitals.
How Splitting Works
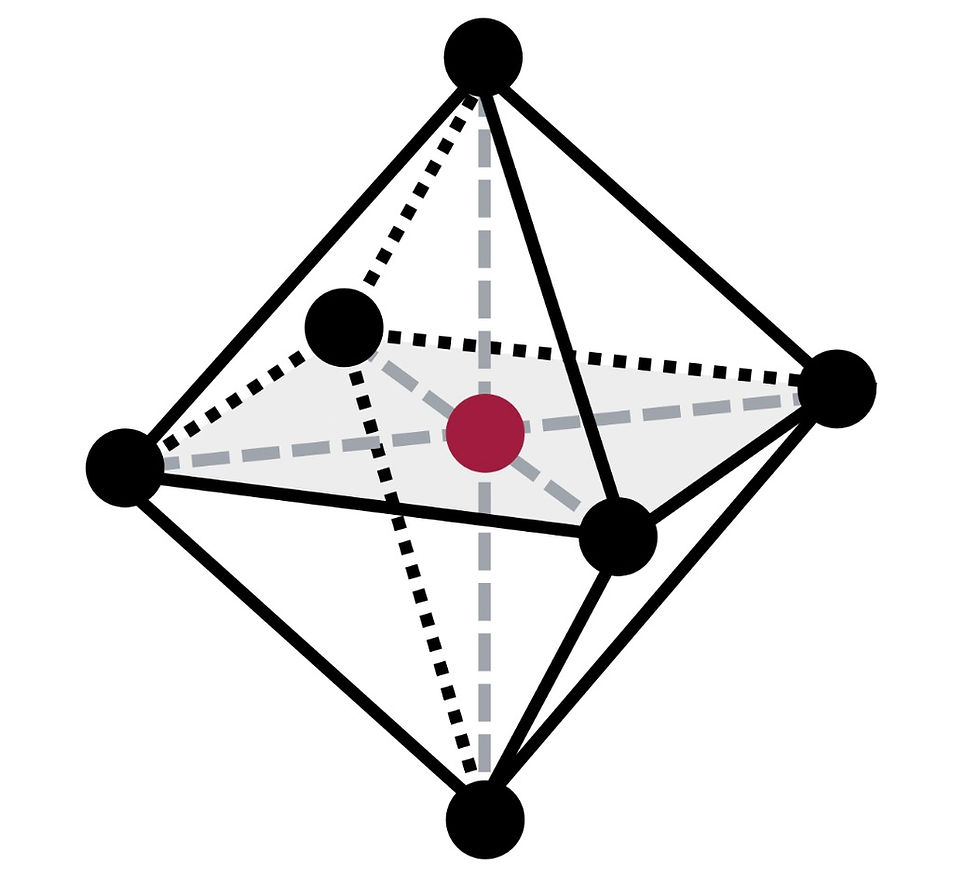
Imagine six ligands surrounding a transition metal ion in an octahedral geometry—with ligands at the six points of an imaginary octahedron. While the d-orbitals of the metal ion experience electrostatic repulsion from the negatively charged electron clouds of the ligands, this interaction isn’t uniform. Orbitals that align directly with the ligands (dz2 and dx2-y2) experience greater electrostatic repulsion and become higher-energy eg orbitals. Orbitals that lie between ligand axes (dxy, dxz, dyz) experience less repulsion and form lower-energy t2g orbitals. The energy difference between these sets of orbitals is called the crystal field splitting energy (Δoct) and is key to a complex’s color.
When white light hits a transition metal complex, electrons in the lower-energy t2g orbitals absorb photons (packets of light) and jump to the higher-energy eg orbitals. The wavelength of light absorbed depends on the size of Δoct, which varies based on the metal ion, its oxidation state, and the ligands attached. The unabsorbed light reflects back to our eyes, creating the color we perceive.
The precise color of a transition metal complex is like a chemical fingerprint, shaped by the identity of the metal ion, its oxidation state, and the nature of its ligands. Each variable contributes to the unique interaction of light with the complex, creating an endless spectrum of possibilities.
Spinning Electrons, Painting the World
From Renaissance paintings to modern dyes, transition metals have transformed the way we see and create color. Transition metals bridge the gap between the microscopic and the macroscopic, turning the subtle movements of electrons into a vivid tapestry of color. So, the next time you marvel at a gemstone or catch the glimmer of a vibrant hue in nature, take a moment to appreciate the chemistry behind it—transition metals spinning their invisible dance to create the visible wonders of our world.
Sources:
Bar-Yosef Mayer, D. E., Vandermeersch, B., & Bar-Yosef, O. (2009). Shells and ochre in Middle Paleolithic Qafzeh Cave, Israel: Indications for modern behavior. Journal of Human Evolution, 56(3), 307–314. https://doi.org/10.1016/j.jhevol.2008.10.005
Domingo, I., & Chieli, A. (2021). Characterizing the pigments and paints of prehistoric artists. Archaeological and Anthropological Sciences, 13(196). https://doi.org/10.1007/s12520-021-01397-y
Elias, M., Chartier, C., Prévôt, G., Garay, H., & Vignaud, C. (2006). The colour of ochres explained by their composition. Materials Science and Engineering: B, 127(1), 70–80. Retrieved from Hal.science
Miessler, G. L., Fischer, P. J., & Tarr, D. A. (2014). Inorganic chemistry (5th ed.). Pearson Education.
Smithsonian Institution's Human Origins Program. (n.d.). Qafzeh: Oldest intentional burial. Retrieved December 21, 2024, from Human Origins website: https://humanorigins.si.edu
Very cool! I have a new apreciation for the colors in nature and art.